
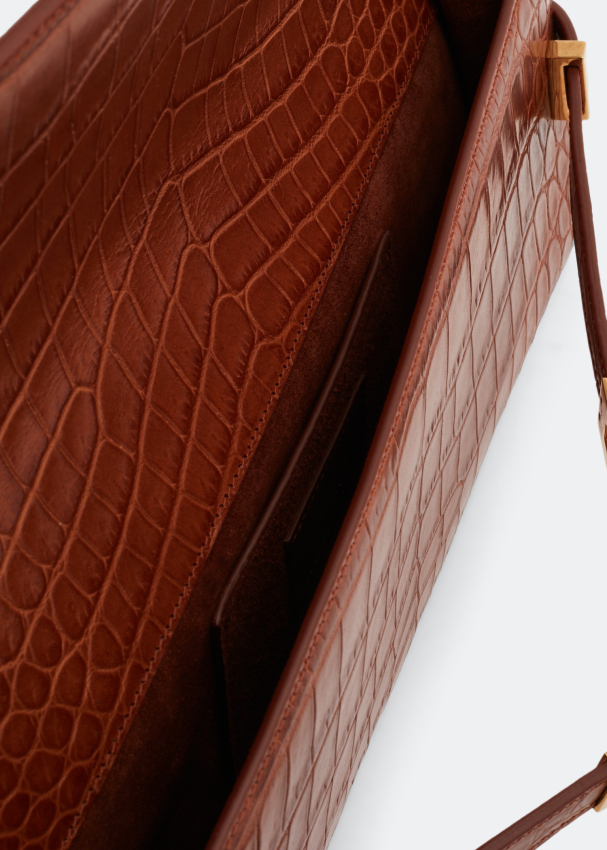
Various high-threshold systems, with diverse applications on more local scales, are reviewed elsewhere, including second-generation so-called underdominant chromosome translocation systems 22 and other strategies such as Medea toxin–antitoxin arrangements 23, 24, 25, 26, 27, 28, 29, Cleave and Rescue 30, 31 or TARE systems 32, in which an essential gene is targeted for inactivation and rescued by a recoded transgene inserted elsewhere in the genome, or reproductive symbionts/parasites such as Wolbachia 33, 34, 35. This Review focuses on the latter low-threshold gene drives in insects as they arguably hold the greatest promise for impacting disease transmission on continental scales and because optimized second-generation drives have been developed over the past 5 years.
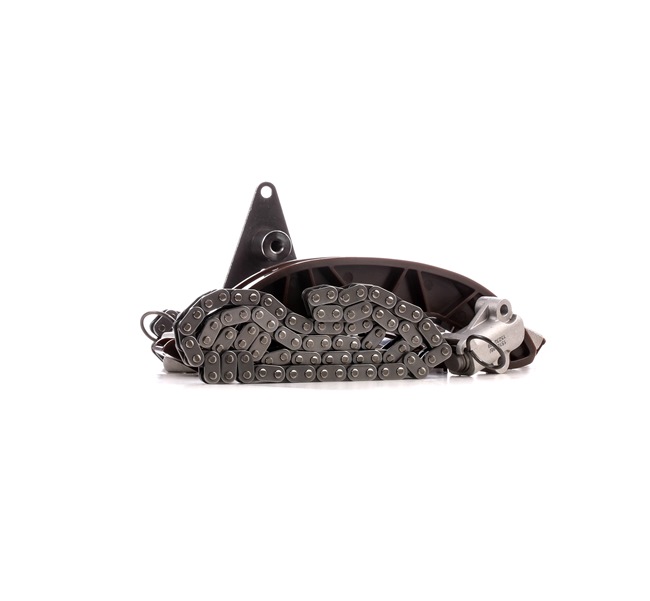
By contrast, low-threshold drives can be seeded at very low numbers to do so. High-threshold drives, such as the reciprocal chromosomal translocations that Curtis considered 2, require many individuals (for example, more than the number of native residents) to take over the population (Fig. Gene drives can be broadly divided into two main categories based on how readily they spread through a population. Such super-Mendelian genetic entities have been implicated in the evolution of genome architectures in plants and animals 17, 18, 19, 20, 21. Driving elements can bias the transmission of sex chromosomes or autosomes (meiotic drive) 4, 5, 6, 7, 8, 9, 10, 11 or only themselves, as exemplified by the diverse families of transposable elements 12, 13 (for example, P-elements in fruitflies 14, 15, 16 or retrotransposons in humans 17). These so-called gene-drive systems or selfish genes 3 are abundant in nature. These scientific advances, combined with ethical and social considerations, will facilitate the transparent and responsible advancement of these technologies towards field implementation.Įxploiting genetic systems that link desired traits to chromosomes or genetic elements with a positive transmission bias (that is, >50%) dates back to the potential uses of chromosomal translocations by Serebrovski 1, which was further generalized and articulated by Curtis in the 1960s for spreading a desired trait throughout a target population 2. This Review summarizes the phenomenal progress in this field, focusing on optimal design features for full-drive elements (drives with linked Cas9 and guide RNA components) that either suppress target mosquito populations or modify them to prevent pathogen transmission, allelic drives for updating genetic elements, mitigating strategies including trans-complementing split-drives and genetic neutralizing elements, and the adaptation of drive technology to other organisms. However, concerns have been raised regarding the potential unintended impacts of gene-drive systems. Recently developed CRISPR–Cas9-based gene-drive systems are highly efficient in laboratory settings, offering the potential to reduce the prevalence of vector-borne diseases, crop pests and non-native invasive species. Gene drives are selfish genetic elements that are transmitted to progeny at super-Mendelian (>50%) frequencies.
